Introduction
Timber is a natural resource that stores carbon, and it can minimize carbon emissions during the construction process [1-7]. Timber absorbs carbon dioxide in the atmosphere during its growth process and stores carbon, thereby fixing carbon in buildings for a long time even after harvesting [8-11]. The United Nations Framework Convention on Climate Change (UNFCCC) also recognizes timber and post-harvest timber products (HWP) as carbon storage, and continuous use of timber is evaluated as a way to promote the regeneration of forest resources and form a virtuous carbon cycle system [12,13].
Overseas, timber construction, which combines new technologies and engineering approaches, is emerging as a sustainable construction solution in modern urban environments [14-20]. Modern timber architecture is attracting attention because of the eco-friendliness of timber as a sustainable building material and the possibility of achieving carbon neutrality [21,22]. Buildings constructed with timber materials have significantly lower carbon emissions during the production and construction process compared to other building materials such as reinforced concrete or steel, thereby reducing the carbon footprint of the entire construction process [23-33]. Several countries, including Canada and Japan, are operating laws and campaigns to encourage the use of timber with the goal of achieving carbon neutrality, and these policies are contributing to the expansion of jobs related to the timber industry [34-38].
In Korea, the expansion of timber construction is also emerging as an important task. The government is promoting the use of timber in public facilities and buildings to achieve the 2050 carbon neutrality goal [39,40]. The Korea Forest Service announced that it is supporting the development of public and private buildings using local timber and discussing laws and guidelines to encourage the preferential use of timber in public buildings [41]. However, Korea has a low self-sufficiency rate of about 16% for timber and is highly dependent on timber imports [42]. In addition, there is a lack of infrastructure for production and processing of timber for building structures. These problems are acting as factors that hinder the growth of the overall timber construction industry [43].
In this study, the domestic and global market trends of timber construction were analyzed from the perspective of timber building materials to understand the status of the domestic timber building industry infrastructure. By understanding the status of the domestic timber building industry infrastructure, we can clearly identify problems and areas in the material supply process that need improvement. This will provide basic data for policy decisions and establishment of industry activation strategies, contributing to increasing the efficiency of the timber supply chain.
Materials and Methods
Fig. 1 shows the research methodology of this study. The materials used in each part of the wooden building, as well as the infrastructure of the wooden building industry, were investigated. In addition, the infrastructure was reorganized from the perspective of materials. The materials and market trends for each part of the wooden building were organized based on a literature review, and a market survey was conducted through interviews with industry professionals.
Fig. 2 shows a virtual timber building designed to illustrate both the exterior and interior of a timber structure. The five sections, roof, connections, wall, floor, foundation, represent the primary structural components of timber buildings. The timber building materials used in these main structural parts were investigated and categorized by material type.
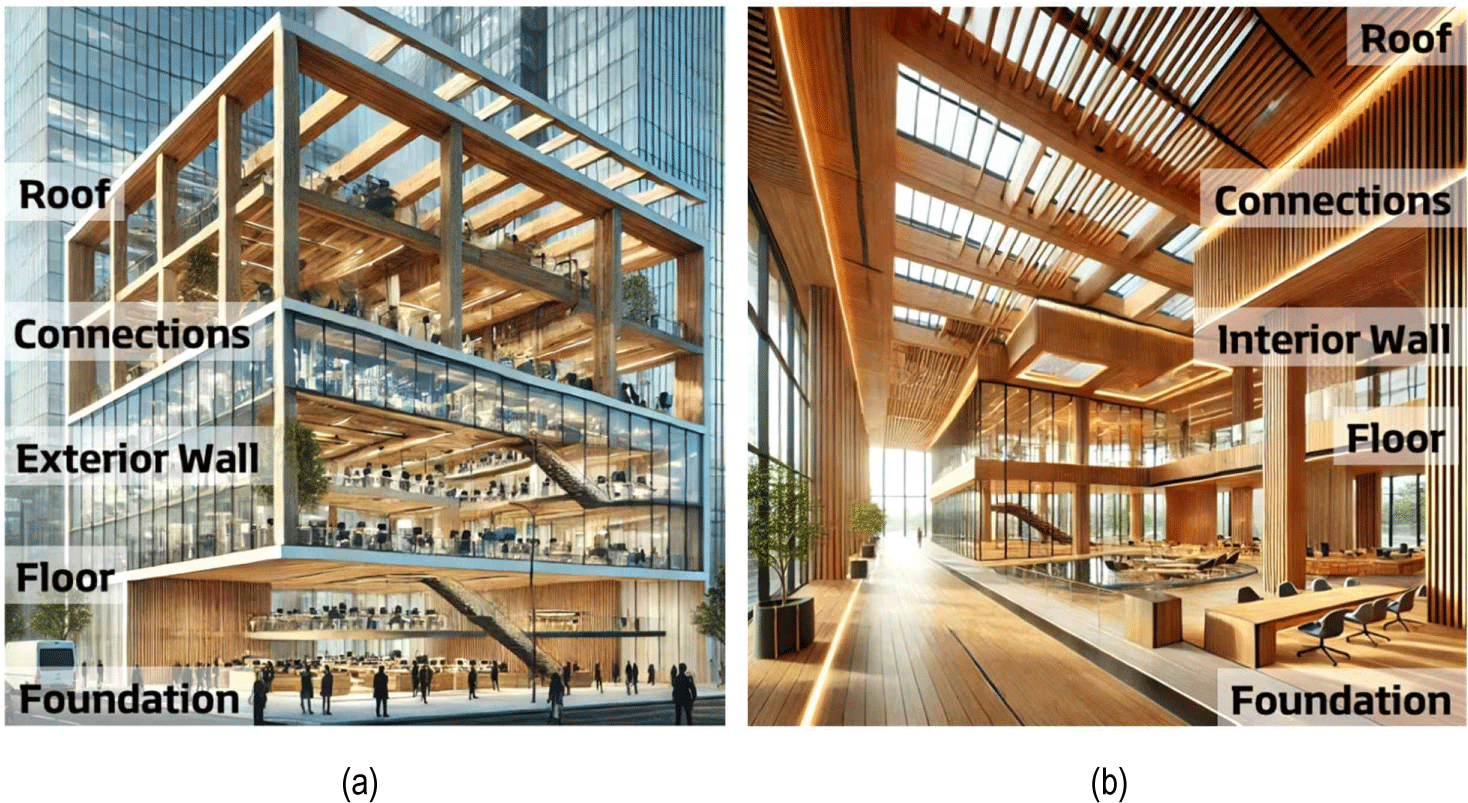
Timber buildings may include structural members such as columns, beams, and trusses, and the structural system can vary based on the arrangement and use of these members. In other words, the same materials may be used for different components, and components may vary depending on the structural system. However, by investigating the five components in Fig. 2, all the components forming the external boundaries (roof, external walls, foundation) and internal boundaries (floor, internal walls, joints) of the timber structure can be reviewed. All the materials of the timber building can be summarized and the overlapping materials can be classified based on the corresponding components.
Results and Discussion
In this study, the main materials used in each major part of the timber structure were analyzed to clarify their characteristics by comparing them with the materials used in reinforced concrete and steel structures. Table 1 summarizes the main materials used in the foundations of timber structures and compares them with those used in reinforced concrete and steel structures. The important factors for each structure are as follows: In timber structures, the foundation requires a waterproofing membrane and moisture management [44]. In reinforced concrete structures, the foundation requires crack management to support the heavy structure [45]. In steel structures, the foundation requires corrosion protection and stable connection through anchor plates. While timber structures use lightweight and shallow foundations, reinforced concrete and steel structures require deeper and stronger foundations due to their heavier loads [46,47]. Each type of foundation has its advantages and is selected based on the building requirements, site conditions, and construction budget.
Table 2 summarizes the main materials used in the floors of timber structures and compares them with those used in reinforced concrete and steel structures. Recently, hybrid structures that take into account the advantages of each material are also being used, and the selection of flooring materials varies depending on the building’s purpose, budget, and environmental conditions [48-50]. Timber floors are lightweight and require moisture management [51-53]. Timber has a natural insulating function, but if higher insulation performance is required, additional insulation is required [54]. Timber floors must be reinforced with fireproof gypsum board or fire retardant treatment for fire resistance [55]. Timber is environmentally friendly and reusable, but is vulnerable to moisture and insects [56].
Reinforced concrete floors are heavy and require strong supports. Although they have excellent soundproofing performance, most concrete apartments in Korea still have problems with inter-floor noise. Steel structure floors are strong and lightweight structures and are used in high-rise buildings and commercial buildings. They have a high possibility of noise transmission, so additional soundproofing materials are required. Steel structures are vulnerable to fire and require fireproofing treatment [57,58]. Steel structures can be recycled, but corrosion management is required [59]. Concrete floors have high carbon emissions and require curing time, but timber floors and steel floors can be constructed quickly using prefabricated components [60,61].
Table 3 summarizes the main materials used in the exterior and interior walls of timber structures and compares them with those used in reinforced concrete and steel structures. Exterior and interior walls have many overlapping items, and most of the contents in Table 3 apply to both exterior and interior walls. Exterior wall materials are required because the wall receives loads and is in contact with the outside, and interior wall materials may also be required for exterior walls in some cases.
Timber structure exterior walls are light and quick to construct; however, timber is vulnerable to moisture and water, necessitating the installation of a moisture barrier [62,63]. Gypsum board and insulation must be added to timber interior walls to reinforce fire resistance and thermal performance. Timber walls are vulnerable to fire, so fireproofing is required [64].
Concrete exterior walls are durable and have excellent soundproofing performance, but they are heavy and require a curing period, making construction time-consuming [65]. Concrete interior walls are structurally sound and have good soundproofing; however, they can generate impact noise [66]. Additionally, concrete walls have excellent fire resistance and durability, but crack management is important, and carbon emissions are high.
Steel frame exterior walls are made of metal panels and glass, making them lightweight and suitable for high-rise buildings. Steel frame interior walls, made of a combination of metal studs and drywall, are lightweight and quick to construct; however, they require additional soundproofing. The metal in steel frame walls is recyclable, but regular maintenance is needed to prevent corrosion and fire, which can be expensive.
Table 4 summarizes the main materials used in the connections of timber structures and compares them with those used in reinforced concrete and steel structures. Connection materials vary depending on the building’s intended use, load requirements, and environmental conditions. In timber construction, nails, screws, bolts, steel plates, and adhesives are primarily used for connections between members. Because these materials are mechanically connected, they provide flexibility and are effective at absorbing vibrations. However, they are vulnerable to moisture and corrosion. Thus, corrosion prevention and regular inspections are necessary.
In reinforced concrete structures, strong connections are formed by combining concrete with embedded steel bars [67]. The connections can support large loads, making them suitable for large and heavy structures. However, they lack flexibility and require ongoing crack management.
In steel structures, connections are typically designed using bolts, welding, anchor plates, and brackets. They have strong resistance to tensile and shear loads and can be constructed rapidly with prefabricated components. Maintenance is required to prevent corrosion and fire damage, and regular inspections are necessary to ensure weld quality.
Table 5 summarizes the main materials used in the roofs of timber structures and compares them with those used in reinforced concrete and steel structures. Timber and steel roofs can be constructed quickly with the use of prefabricated components. Concrete roofs require curing, so construction time is longer. Timber is the most environmentally friendly, while concrete has a high carbon footprint, and steel is recyclable but requires corrosion management [68].
Timber roofs are suitable for housing because of their light weight and natural insulation, and they are also used for large building roof structures, such as gymnasiums, auditoriums, train stations, and airports, because of their excellent strength-to-weight ratio. Timber roofs can be constructed quickly, but require regular moisture management.
Concrete roofs are suitable for commercial buildings and infrastructure due to their durability and fire resistance. However, they require inspection to prevent cracks, and insulation reinforcement is needed because of their poor insulating properties. Steel roofs are suitable for industrial facilities and high-rise buildings. They can be constructed quickly, but require corrosion protection and fire management. Fireproof coating is essential.
Table 6 reorganizes the contents of Tables 1 through 5 by material and adds information major manufacturers. It categorizes wood-based and non-wood-based materials, summarizing the components used in timber construction and their major manufacturers. This table clearly shows the corresponding components by material, facilitating efficient procurement.
Category | Material | Application | Manufacturer | |
---|---|---|---|---|
Domestic | Overseas | |||
Wood-based materials | Cross-laminated timber (CLT) | Floor, walls, roof | KMbeam [69], Huin [70], HCwood [71], SYwood [72], DHwood [73], National Forestry Cooperatives Federation [NFCF] [74] | Stora Enso [86], Binderholz [87], KLH [88] |
Glue laminated timber (Glulam) | Structural column & beams, roof | KMbeam [69], Huin [70], HCwood [71], SYwood [72], DHwood [73], National Forestry Cooperatives Federation [NFCF] [74] | Hasslacher Norica Timber [89] | |
Laminated veneer lumber (LVL) | Structural beams, floor, walls, roof | None | Boise Cascade [90], Carter Holt Harvey [91], Weyerhaeuser [92] | |
Oriented strand board (OSB) | Walls, floor, roof | None | Norbord (West Fraser) [93], Kronospan [94], Egger [95] | |
Plywood | Walls, floor, roof | Sungchang Enterprise [75], Sun&L [76], Eagon Industrial [77] | UPM Plywood [96], Greenply [97], Georgia-Pacific [98] | |
Particleboard | Walls, floor, roof | Dongwha [78] | Egger [95], Sonae Arauco [99], Swiss Krono [100] | |
Solid timber (studs, joists, rafters) | Wall, floor, roof | 1,149 companies [79] | Too many to enumerate | |
Non-wood-based materials | Concrete | Foundation | Namyang Construction [80], Daehan Materials [81] | Holcim [101], CEMEX [102], HeidelbergCement [103] |
Waterproof membrane | Foundation, wall, roof | KCC [82], Hyundai Construction [83] | Sika [104], GCP Applied Technologies [105], Carlisle [106] | |
Steel plate | Connections | Megatie [84] | Rothoblaas [107], Simpson Strong-Tie [108], Nippon Steel [109] | |
Metal fasteners | Connections | Megatie [84] | Rothoblaas [107], Simpson Strong-Tie [108], Hilti [110], Sherpa [111] | |
Gypsum board | Walls, floor | KCC [82], LG Hausys [85] | USG [112] | |
Adhesives | Connections and structural reinforcement | None for structural timber | Henkel [113], 3M [114], Sika [104] | |
Insulation materials | Walls, floor, roof | KCC [82], LG Hausys [85] | Rockwool [115], Owens Corning [116], Knauf Insulation [117] , CTBUH [118] |
Wood-based materials such as cross-laminated timber (CLT), glued-laminated timber (GLT), laminated veneer lumber (LVL), oriented strand board (OSB), and particle board are used for structural elements like floors, walls, and roofs (Fig. 3). These materials are well-suited for modern timber construction due to their excellent structural performance and environmental sustainability (Fig. 4). CLT is used in mid- and high-rise timber buildings, and in Korea, domestic companies such as Kyungmin Industries (kmbeam), Huin, and National Forestry Cooperatives Federation (NFCF) produce it. Globally, companies like Stora Enso and KLH, which use automated production systems, supply CLT to the international market. In contrast, domestic manufacturers use semi-automated systems and produce based on orders. Although their production volumes are smaller compared to automated overseas manufacturers, domestic manufacturers have the technology to produce structural CLT using local wood, allowing for customized production that meets consumer specifications and quality requirements.
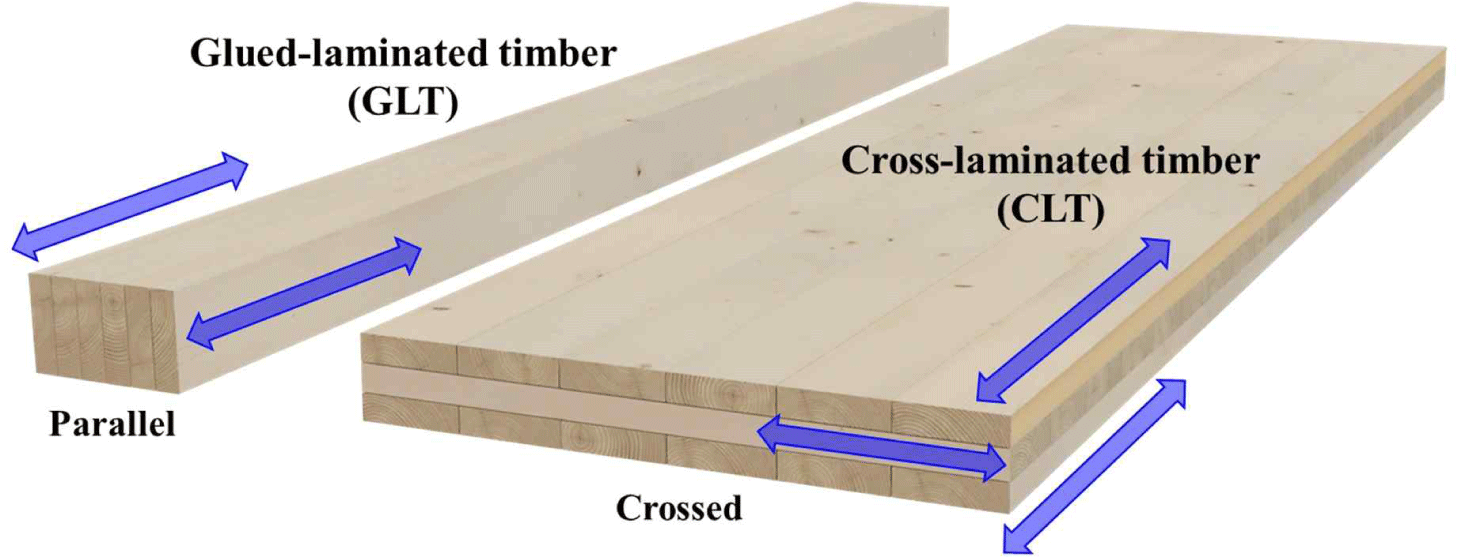
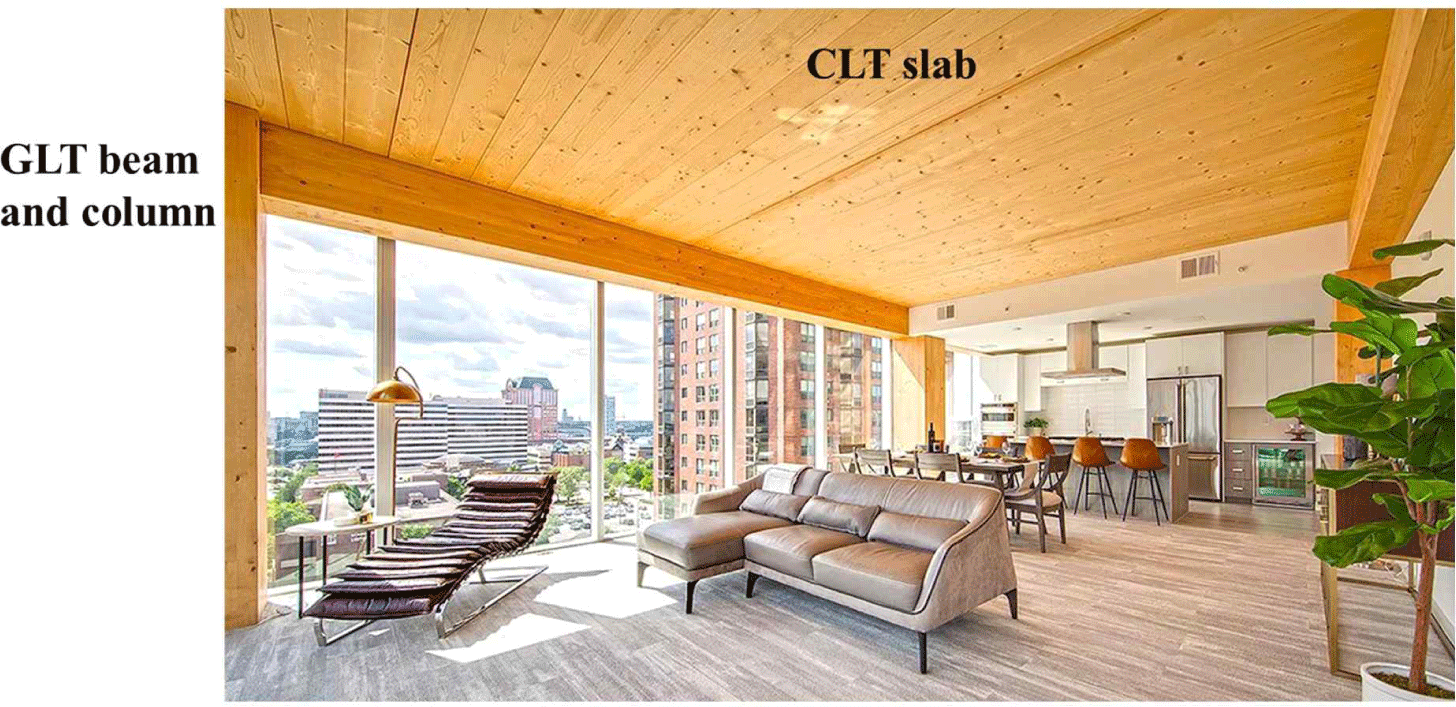
Domestic CLT manufacturers typically also undertake construction. CLT and structural laminated lumber are used for key structural components and roofs, with six manufacturers currently operating in Korea. These manufacturers use facilities originally designed for laminated lumber or plywood, allowing them to produce CLT panels without length restrictions. However, the panel width is limited to less than 1.2 m. Initially, resorcinol adhesives were commonly used, but there is now a trend toward eco-friendly PUR or isocyanate adhesives, which do not contain formaldehyde (Table 7).
Representative non-wood-based materials include concrete, steel plates, and insulation, which enhance structural stability and energy efficiency. In timber construction, timber members are generally connected using steel products. In other countries, various steel connectors for timber have been developed and commercialized. In Korea, companies like POSCO, Hyundai Steel, and Dongkuk Steel lead the global steel industry, indicating the potential for Korea to lead the global market for timber construction connection hardware through research and technological advancements.
Gypsum board and insulation are used to ensure fire resistance and thermal performance in interior walls and floors. Global manufacturers such as KCC, LG Hausys, and Eagon Industrial are based in Korea. Adhesives also play a crucial role in reinforcing connections and assembling structures. Korea’s petrochemical industry, a core sector of the national economy, is highly competitive both regionally and globally. This advantage enhances the competitiveness of domestic modern timber construction and its potential for growth into a new industry.
Fig. 5 shows the number of buildings under construction and the total floor area of timber buildings in South Korea over the past 20 years. The blue bar represents the number of buildings under construction, which increased over time, peaked in 2016, and then declined. Since 2019, approximately 10,000 units have been constructed annually. The orange line depicts the total floor area of timber buildings, which followed a similar trend. It increased until 2016, then declined, and has remained steady at around 1 million m2 since 2019. Currently, most of the domestic wooden building market consists of single-family houses, and overall market growth has stagnated. Therefore, there is a need to create new markets to expand timber building construction and boost timber consumption.
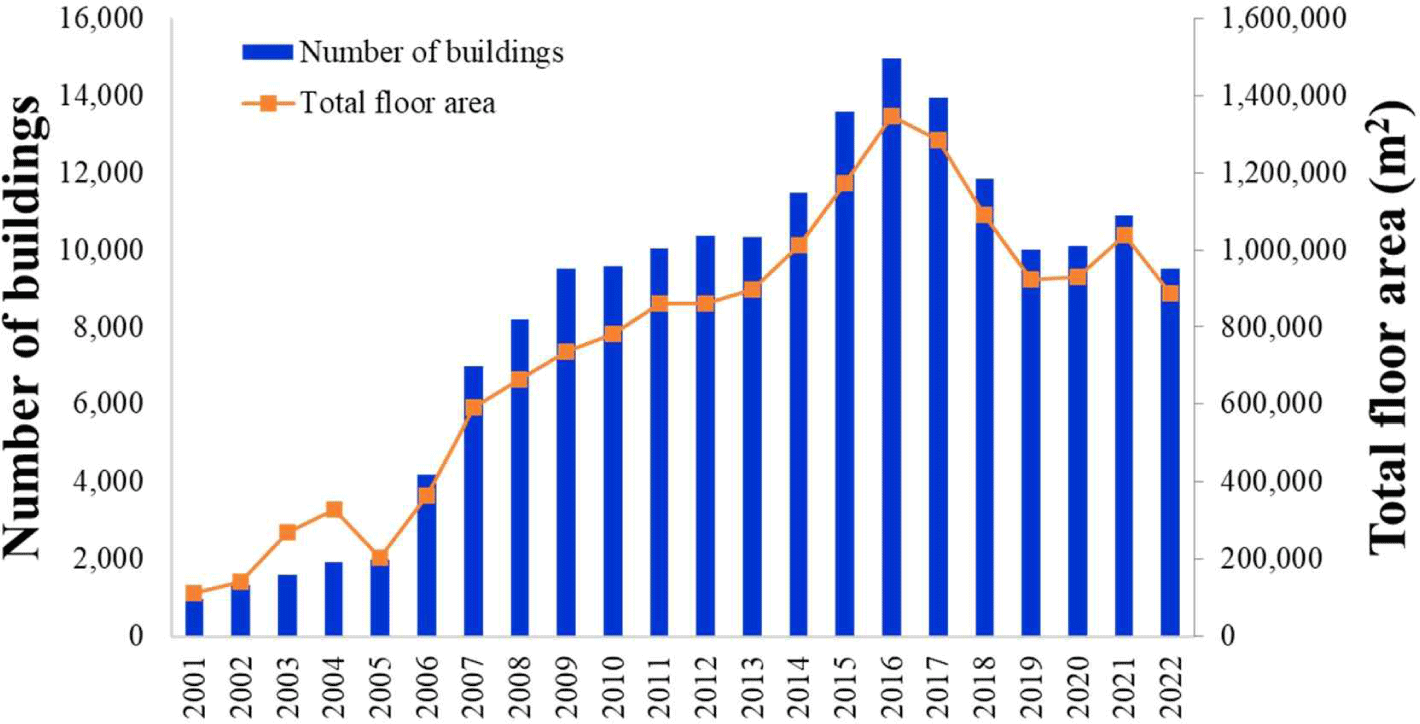
The Council on Tall Buildings and Urban Habitat 64118 provides the data for timber buildings over 8 stories tall, built worldwide since 2008. Fig. 6 summarizes images of representative high-rise timber buildings and key information about them. HoHo Timber is an 84-meter-tall, 24-story timber tower located in Vienna, Austria. Completed in 2019, it is a symbolic example of the potential of timber construction.
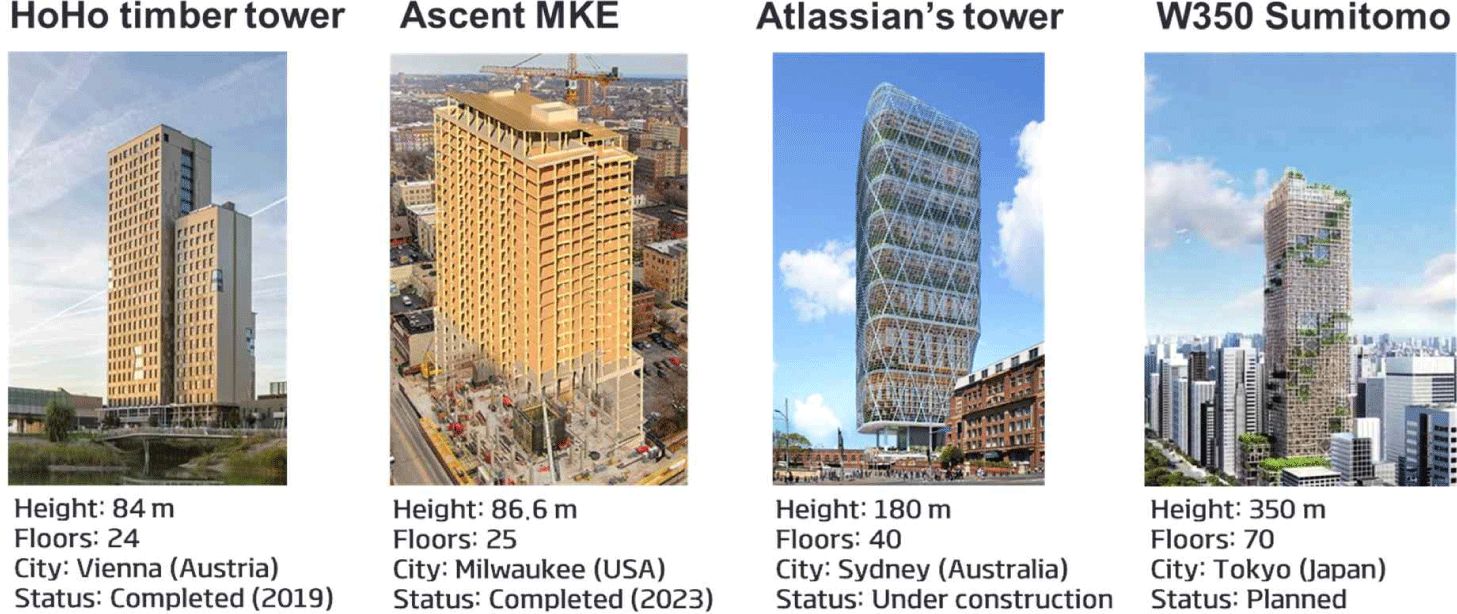
Ascent MKE is an 86.6-meter-tall, 25-story building located in Milwaukee, USA, which was completed in 2023. Currently the tallest timber building, it is another example of the advancement of modern timber construction technology, demonstrating the potential of timber buildings, especially in North America.
Atlassian’s Tower is a 180-meter-tall, 40-story building currently under construction in Sydney, Australia. This building is a supertall structure that uses timber as its primary material and is expected to be one of the tallest timber buildings in the world when completed.
W350 Sumitomo is a 350-meter-tall, 70-story timber building planned for Tokyo, Japan. Still in the planning stages, this building is expected to be the world’s tallest timber structure when completed and will set a new standard for eco-friendly construction by maximizing the use of wood.
Fig. 7 is a graph visualizing the change in the number of timber buildings over 8 stories tall and their maximum height, built worldwide since 2008. The blue bars represent the number of buildings by year, and the orange line represents the maximum height of timber buildings built during that period. The number of timber buildings over 8 stories has increased over time, and their height has also increased.
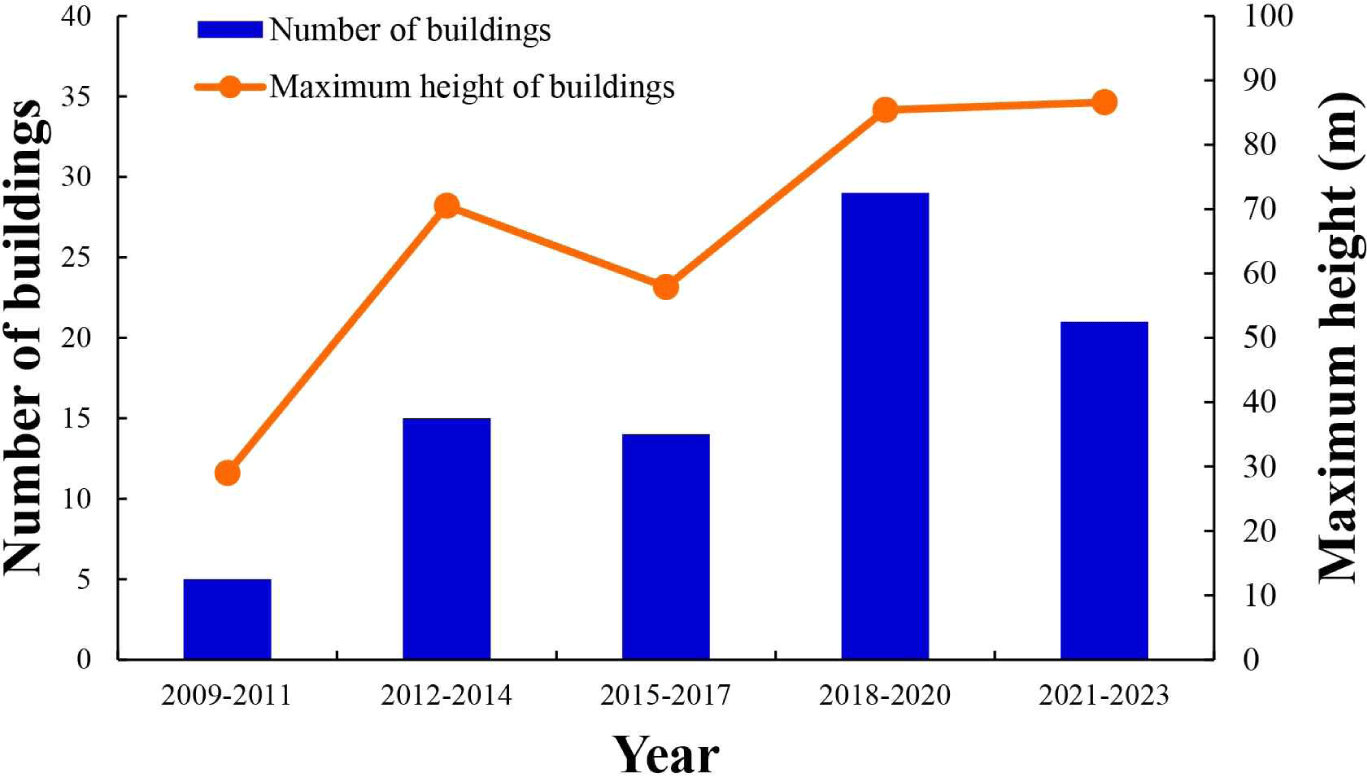
Several researchers have investigated the timber construction and wood products market. Albee (2019) reported that the adoption of CLT in commercial and industrial construction is increasing in North America and Europe. In North America, the use of CLT is expanding in public libraries, university housing, and similar projects. As a result, the global CLT market size is expected to more than double from $664 million in 2018 to $1.457 billion in 2024.
Nepal et al. (2021) also noted that regulations on timber construction are being relaxed in North America, leading to increased use of CLT in large public buildings and high-rise buildings. Specifically, the demand for large wood products such as CLT is rising, with a global production volume of 2.8 million m3 recorded in 2020. The CLT market is projected to grow continuously from 2021 to 2027 at a compound annual growth rate of 12%–14%.
In Korea, the regulation limiting the roof height of timber buildings to 18 meters was removed in November 2020 as part of the revisions to structural standards for buildings. This change makes it possible to construct mid-rise timber buildings in cities, enhancing their competitiveness. If mid-rise timber buildings become more common in cities, the currently stagnant timber building market, as shown in Fig. 5, is expected to grow again. Demand for high-performance wood products such as CLT and timber building materials will also increase. Timber buildings provide a means of long-term carbon storage and will contribute to achieving carbon neutrality in the construction industry.
Conclusion
-
Timber construction materials and timber structures are lightweight, allowing for shallow foundations, and have the flexibility to effectively absorb vibrations. Timber construction materials such as CLT and LVL offer excellent structural performance, faster construction speed, and a lower carbon footprint, making them environmentally friendly. In contrast, reinforced concrete and steel structures require heavier and stronger foundations but provide greater durability and fire resistance.
-
Analysis of the infrastructure for timber building materials shows that Korea has a low self-sufficiency rate in wood and lacks infrastructure, but domestic manufacturers have the technology to produce high-quality wood products using domestic wood. Semi-automatic systems enable customized production to meet consumer needs.
-
The domestic timber construction market in Korea has primarily consisted of single-family homes and has remained stagnant since 2016, with an annual market size of about 10,000 units. However, the recent removal of height restrictions on timber buildings has created opportunities for mid-rise and high-rise timber construction in urban areas. This regulatory change is expected to boost demand for high-performance wood products like CLT, potentially revitalizing the domestic timber construction market and fostering growth in the wood industry.
-
This study focuses on the material and infrastructure aspects of timber construction. Future research should explore the carbon storage and offset effects of timber construction, its contribution to carbon neutrality, and its socio-economic and policy implications. Advanced manufacturing technologies, such as automated production lines, should also be developed to improve the production efficiency and quality of engineered wood products like domestic CLT. Additionally, detailed studies on structural optimization, fire safety, and acoustic performance are essential for the successful adoption of mid- and high-rise timber construction in cities. These efforts will support the sustainable growth of the timber construction industry.