서 론
산업의 발달로 석유, 석탄 등의 화석연료에 대한 의존도가 증가하면서 지구온난화에 의한 기후변화가 심각해지고 있다. 이러한 문제를 해결하기 위해서는 화석연료를 대체하는 지속가능한 에너지 기술 개발이 필요하다[1]. 바이오매스는 재생 가능하고 탄소 중립적이며 화석연료를 대체할 수 있는 에너지원으로 다양한 공정을 통해 바이오에너지, 기능성 화학물질 등으로 전환할 수 있다[2]. 바이오매스는 셀룰로오스, 헤미셀룰로오스 및 리그닌으로 구성된 견고한 세포벽 구조를 가지고 있는 난분해성 소재로 바이오매스의 구성성분을 활용하기 위해서는 세포벽 구조를 파괴하기 위한 전처리 과정이 필요하다.
전처리 방법으로 물리적, 화학적, 물리 화학적 방법이 있다. 물리적 방법에는 볼밀, 초음파, 압출 등이 있다. 물리적 전처리는 결정화도 및 입자크기를 감소시키며 바이오매스 내부 구조를 파괴하여 효소가수분해 효율을 향상시킨다[3]. 하지만 물리적 전처리는 많은 에너지가 소비되는 단점이 있다. 화학적 전처리는 산, 알칼리, 유기용매 등의 화학물질을 활용하여 바이오매스의 구성성분을 분해하여 효소가수분해 효율을 향상시킬 수 있다. 그러나 화학물질 사용으로 인한 환경 오염문제, 공정의 어려움 등의 단점이 있다[4]. 물리·화학적 전처리에는 수열처리, 마이크로파 처리 등이 있다. 수열처리는 160℃–240℃에서 H2O를 사용하여 바이오매스를 분해하는 공정으로 화학약품을 사용하지 않아 친환경적이다. 수열처리 중 H2O에서 생성된 H3O+가 헤미셀룰로오스 분해에 관여하여 헤미셀룰로오스가 선택적으로 분해된다. 따라서, 효소가수분해 과정에서 셀룰로오스에 대한 효소의 접근성이 향상되어 효소가수분해 효율을 향상시킨다[1]. 하지만 바이오매스의 난분해성 구조 때문에 단일 전처리로 높은 효소가수분해 효율을 얻기에는 한계가 있다. 물리적 전처리 방법 중 볼밀은 바이오매스의 입자크기를 감소시켜 효소가 접근 가능한 표면적을 증가시키고 셀룰로오스의 결정성을 감소시켜 효소가수분해 효율을 향상시킬 수 있다. 따라서 수열처리와 볼밀 전처리의 연속 공정은 기존 전처리의 단점을 극복할 수 있다[5].
수열처리는 고온·고압에서 수행되기 때문에 전처리 과정에서 다량의 증기가 발생한다. 이러한 증기를 응축한 응축수에는 바이오매스 분해산물인 당 분해 물질과 리그닌 분해 물질을 포함하고 있다[6]. 일반적으로 수열처리에서 발생하는 증기는 공기중에 배출되어 재활용되지 못하고 있다. 하지만, 응축수에는 다양한 유기산을 포함하고 있어 수열처리 공정에서 H2O 대신 사용할 경우, 전처리 효율 향상을 기대할 수 있다[7].
거대억새(miscanthus, M)는 생장 기간이 짧고, 수확량과 셀룰로오스 함량이 높다[8]. 단수수는 짧은 생장 기간(3–5개월), 뛰어난 가뭄 내성, 당 함량이 높으며, 즙을 추출한 후 발생하는 단수수 바가스(sweet sorghum bagasse, S)는 바이오에너지 생산 원료로 널리 활용되고 있다[9]. 참나무(Oak, O)는 생장이 빠르고 자원이 풍부하며, 활엽수 중에서 아세틸기를 많이 함유하고 있는 소재로 수열처리 과정에서 자기가수분해 효과가 높다[10].
본 연구에서는 바이오매스(거대억새, 단수수 바가스, 참나무)의 전처리로 볼밀과 수열처리를 수행하였으며, 전처리 및 효소가수분해 효율을 향상시키기 위해 수열처리에 H2O 대신 응축수를 사용하였다. 전처리 바이오매스의 특성과 효소가수분해 효율을 비교하여 효율적인 전처리 조건을 탐색하였다.
재료 및 방법
본 연구에서는 거대억새(miscanthus, M), 단수수 바가스(sweet sorghum bagasse, S), 참나무(Oak, O)를 공시재료로 사용하였다. 거대억새와 단수수 바가스는 한국화학연구원 바이오화학연구센터에서 제공받았으며, 참나무는 경북 구미시에서 구매하여 사용하였다. 바이오매스는 분쇄기를 이용하여 20–80 mesh로 분쇄하였으며, 함수율은 10% 이내를 유지하였다.
바이오매스의 구성성분은 NREL(National Renewable Energy laboratory) 방법으로 분석하였다[11]. 바이오매스 수열처리 응축수는 한국화학연구원 바이오화학연구센터에서 제공받았으며, 응축수 구성성분은 HPLC(Waters 2695, Waters, USA)를 이용하여 분석하였다. 컬럼으로 Aminex HPX-87H column(300×7.8 mm, Bio-Rad, USA), 검출기는 Refractive index detector(Waters 2414, Waters)를 사용하였다. 이동상으로 5 mM H2SO4를 0.6 mL/min의 속도로 흘려주고 55분 동안 분석하였다. 응축수 성분분석 결과는 Table 1에 나타냈다.
바이오매스와 증류수(또는 응축수)를 1:10(W/W)의 비율로 혼합하여 고온·고압 반응기(EMW-HT/HP600-3, EMS, Korea)에 넣고 170℃에서 10분, 252×g의 속도로 수열처리 하였다. 반응 후 액상가수분해산물과 고형 바이오매스를 분리하였으며, 고형 바이오매스의 구성성분 및 액상가수분해산물 분석은 2항의 방법에 따라 분석하였다. 볼밀 처리는 바이오매스 25 g과 지르코늄 ball(ф=10 mm) 530 g을 반응기에 넣고 Planetary mill(PM 100, Retsch, Germany)을 이용하여 2,263×g의 조건에서 10분 동안 처리하였다.
바이오매스의 결정화도는 3D 고분해능 X선 회절분석기(X-ray diffractometer: XRD, EMPyrean, PANalytical, Netherlands)를 이용하여 2θ=5–90°, 60 kV, 55 mA의 조건에서 분석하였다. 결정화도는 Segal법에 따라 계산하였다[12].
바이오매스 구조의 변화는 푸리에 변환 적외선 분광기(Fourier-transform infrared spectrometer: FT-IR, Spectrum 3, PerkinElmer, USA)를 이용하여 4,000–560 cm–1의 범위에서 4 cm–1의 해상도로 16회 측정하여 분석하였다.
바이오매스 2 g과 sodium citrate buffer(50 mM, pH 4.8) 20 mL를 250 mL 삼각플라스크에 넣고 혼합한 후, 당화효소(Cellic CTec3 HS, Novozymes, Denmark) 26.4 FPU/g을 첨가하여 효소가수분해를 수행하였다. 반응온도 50℃에서 113×g으로 72시간 동안 수행하였으며, 24시간 간격으로 시료를 취하여 glucose 함량을 HPLC로 분석하였다. HPLC 분석조건은 2항과 같다.
바이오매스의 효소 흡착 등온선을 결정하기 위한 흡착 실험은 다음과 같다. 바이오매스와 50 mM citrate buffer(pH 4.8)를 1:10(W/V) 비율로 혼합한 후, 당화효소(Cellic CTec3 HS, Novozymes, Denmark)를 5–20 FPU/g 첨가하여 반응온도 50℃에서 88×g으로 2시간 동안 흡착실험을 수행하였다. 이후, 액상은 12,879×g으로 10분간 원심 분리하여 상등액의 단백질 함량을 측정하였다. 단백질 함량은 Bradford 방법으로 측정하였으며, 상등액과 Coomassie brilliant blue G-250를 혼합하여 10분 반응한 후, UV-vis spectrometer(UV-1800, Shimadzu, Japan)를 이용하여 595 nm에서 측정하였다[13].
결과 및 고찰
바이오매스의 성분분석 결과는 Fig. 1a에 나타냈다. 모든 바이오매스에 대해 수열처리를 수행한 결과, 주로 헤미셀룰로오스가 분해되었으며, 바이오매스에 포함된 헤미셀룰로오스의 함량은 13.64%–16.82%였다(원시료: 24.39%–32.4%). 이것은 수열처리의 전형적인 특징으로, H2O의 이온화로 생성된 하이드로늄 이온(H3O+)에 의한 헤미셀룰로오스의 선택적 제거 때문이다[14]. 응축수를 사용한 수열처리에서 바이오매스의 헤미셀룰로오스 분해는 촉진되었으며, 헤미셀룰로오스 함량은 감소하였다(11.56%–14.58%). 응축수 종류에 따른 차이는 거의 없었다. 반면, 셀룰로오스의 함량은 증가하였는데, 이것은 헤미셀룰로오스 제거에 의한 상대적인 증가이다. 응축수에 포함된 유기산(아세트산, 포름산 등)은 하이드로늄 이온과 함께 산전처리 효과를 유도하여 헤미셀룰로오스 분해를 촉진하였다[15]. 응축수의 pH는 2.85로 약산성을 나타내고 있어 H2O(pH 6.09)와 비교하여 충분히 산전처리의 효과를 기대할 수 있다. 특히, 응축수에 포함된 아세트산은 수열처리 과정에서 헤미셀룰로오스 분해로 생성된 대표적인 유기산으로 바이오매스 자기가수분해에서 산 촉매로 작용한다[15]. 이러한 결과는 중량감소율과 액상가수분해산물 분석결과로 확인할 수 있다(Fig. 1b). 모든 바이오매스에서 응축수를 사용한 전처리에서 높은 중량감소율을 나타냈으며(hydrothermal pretreatment using condensate of oat bran [OCHT]: 15.96%–30.22%, hydrothermal pretreatment using condensate of sweet sorghum bagasse [SCHT]: 14.77%–30.37%), 액상가수분해산물에 포함된 구성성분의 함량도 증가하였다. 하지만, 응축수 종류에 따른 차이는 거의 없었다. 모든 수종에서 수열처리 후 볼밀을 수행한 결과, 바이오매스 구성성분의 차이는 거의 없었다. 물리적 전처리는 바이오매스 구성성분을 분해 또는 전환하지 못하는 것을 의미하며, 기존 연구결과와 일치한다[16]. 하지만 수열처리 후 볼밀은 바이오매스의 입자크기 및 결정화도를 감소시켜 단일전처리 보다 효소가수분해 효율을 향상시킬 수 있다[17]. 바이오매스의 헤미셀룰로오스는 셀룰로오스의 미세 섬유를 덮고 있어 효소가 셀룰로오스에 접근하는 것을 방해하는 물리적 장벽이다. 따라서, 헤미셀룰로오스는 효소가수분해 효율을 저해하는 요인 중 하나이다[18]. 따라서 전처리를 통해 바이오매스의 헤미셀룰로오스가 제거됨에 따라 효소가수분해 효율 향상을 기대할 수 있다.
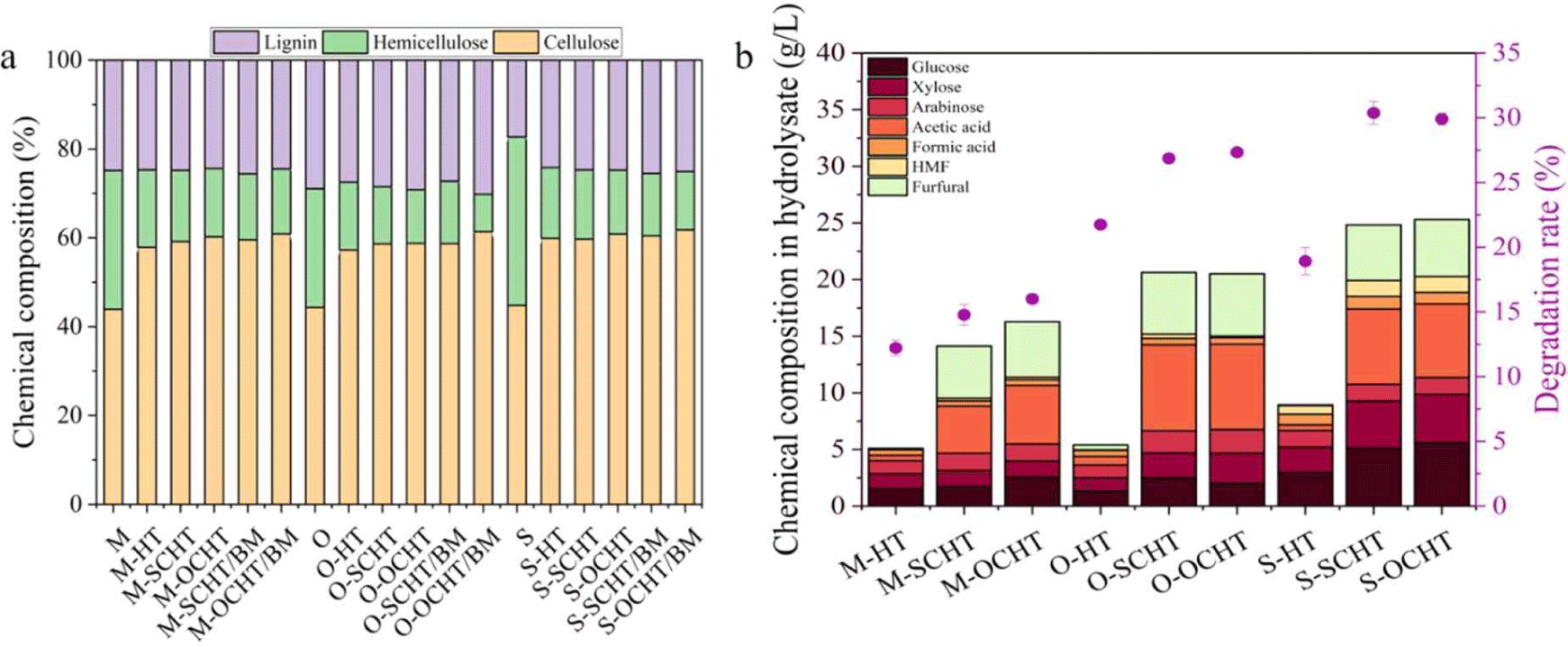
바이오매스의 결정화도 분석 결과는 Fig. 2a–2c에 나타냈다. 바이오매스 종류에 따라 결정화도의 차이가 있었으며, 이것은 구성성분 함량의 차이 때문이다. 바이오매스와 응축수 종류와 상관없이, 응축수 전처리 바이오매스의 결정화도가 원시료보다 높게 나타났다. 응축수 전처리는 기존의 수열처리보다 낮은 pH 조건에서 수행되어 주로 헤미셀룰로오스와 비결정성 셀룰로오스가 분해된다. 따라서 상대적으로 결정성 셀룰로오스가 증가하여 결정화도가 증가하였다. 반면, 응축수/볼밀 전처리 바이오매스의 결정화도는 감소하였다. 셀룰로오스 관련 피크(2θ=16, 22°)의 강도가 감소하여 SCHT/BM에서 12.00%–39.56%, OCHT/BM에서 10.11%–39.22%로 응축수 전처리(52.84%–61.44%) 및 수열처리(50.58%–54.28%) 바이오매스와 비교하여 크게 감소하였다. 이것은 볼밀 과정에서 볼과 바이오매스 간의 마찰로 인해 바이오매스의 결정성 셀룰로오스가 파괴되어 나타난 결과이다[19]. 따라서, 셀룰로오스의 노출로 효소가 접근할 수 있는 비표면적을 증가시켜 효소가수분해 효율 향상을 기대할 수 있다.
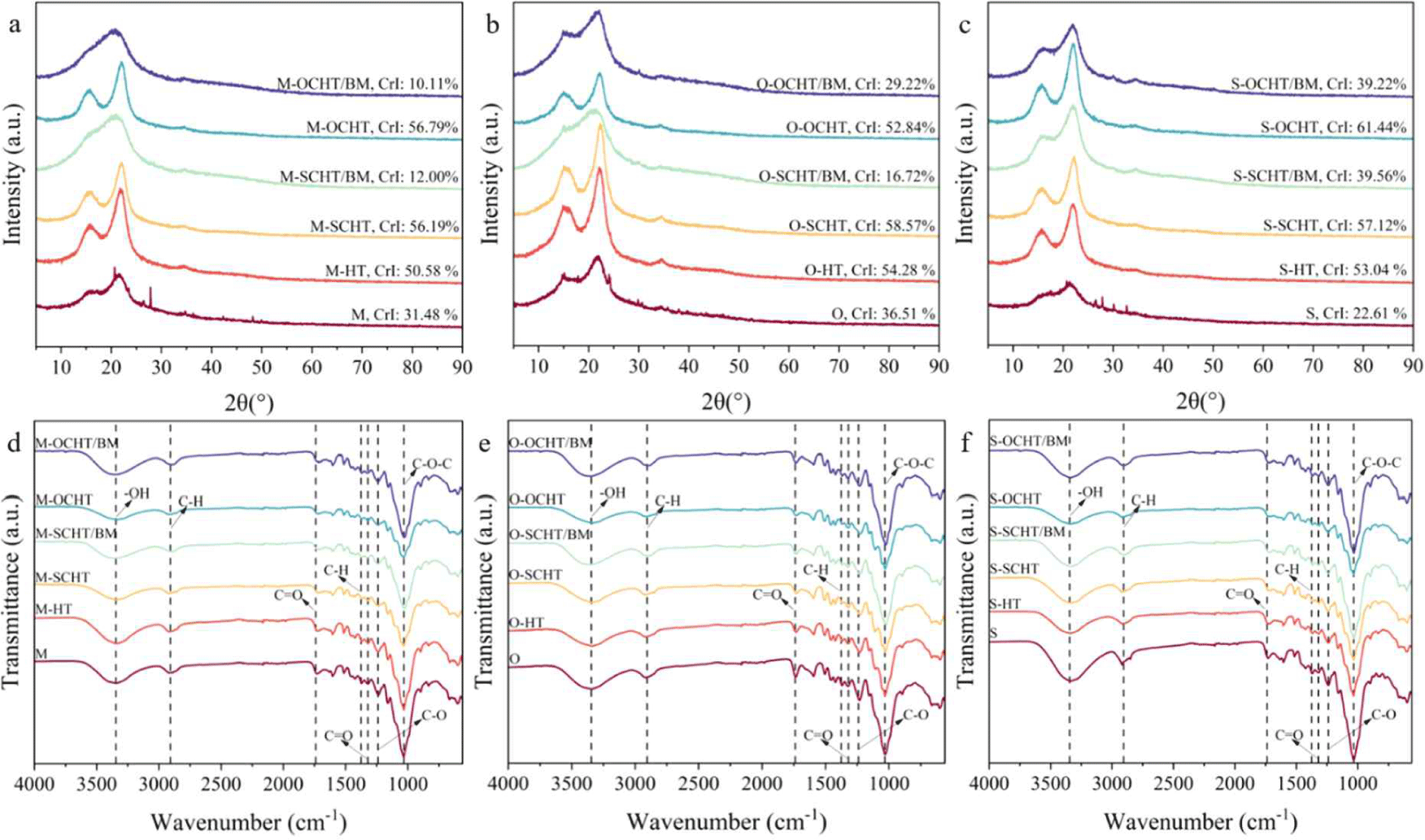
전처리에 의한 바이오매스 구조변화는 FTIR 분석으로 확인하였으며, 결과는 Fig. 2d–2f에 나타냈다. 3,345 cm–1(O-H stretching), 2,906 cm–1(C-H stretching), 1,055 cm–1(C-O-C)에서 셀룰로오스 관련 흡수 피크가 나타났다[20]. 헤미셀룰로오스 관련 피크는 1,739 cm–1(C=O stretching), 1,376 cm–1(C-H aliphatic stretching), 1,240 cm–1(C-O stretching)에서 나타났으며 응축수/볼밀 전처리 바이오매스에서 피크의 강도가 감소하였다. 이것은 헤미셀룰로오스가 분해되어 나타난 결과이다. 리그닌 관련 피크는 1,320 cm–1(C=O stretching)에서 나타났으며, 전처리에 따른 피크 강도의 변화는 거의 없었다. 따라서, 응축수 전처리에 의해 헤미셀룰로오스 분해가 촉진된 것을 확인하였으며, 이 결과는 화학성분 분석결과와 일치한다(Fig. 1).
바이오매스의 효소가수분해 결과는 Fig. 3a–3c에 나타냈다. 수열처리, 응축수 전처리, 응축수/볼밀 전처리 바이오매스의 효소가수분해 효율(셀룰로오스에서 글루코오스 전환율)은 시간에 따라 점진적으로 증가하였다. 응축수 전처리 바이오매스의 효소가수분해 효율은 SCHT에서 91.38%–93.90%, OCHT에서 94.06%–96.78%로 수열처리 바이오매스(29.92%–57.83%) 대비 높게 나타났다. 응축수 전처리에 의해 헤미셀룰로오스 분해가 촉진되어 효소가수분해 효율을 향상시킨 것이다. 응축수 종류에 따른 차이는 거의 없었다. 응축수/볼밀 전처리로 SCHT/BM에서 96.57%–98.13%, OCHT/BM에서 98.32%–99.07%로 응축수 전처리 바이오매스와 비교하여 효소가수분해 효율이 향상되었다. 이것은 볼밀 전처리 과정에서 바이오매스 입자 크기가 감소하고 셀룰로오스의 결정성 영역의 감소로 효소의 셀룰로오스 접근성이 향상되어 효소가수분해 효율이 증가한 것으로 판단된다[3]. 응축수 종류에 따른 효소가수분해 효율 차이는 크지 않았다. 두 종류의 응축수에 포함된 유기산은 바이오매스 전처리에서 산 촉매 역할을 충분히 하여 효소가수분해 효율을 향상시켰다.
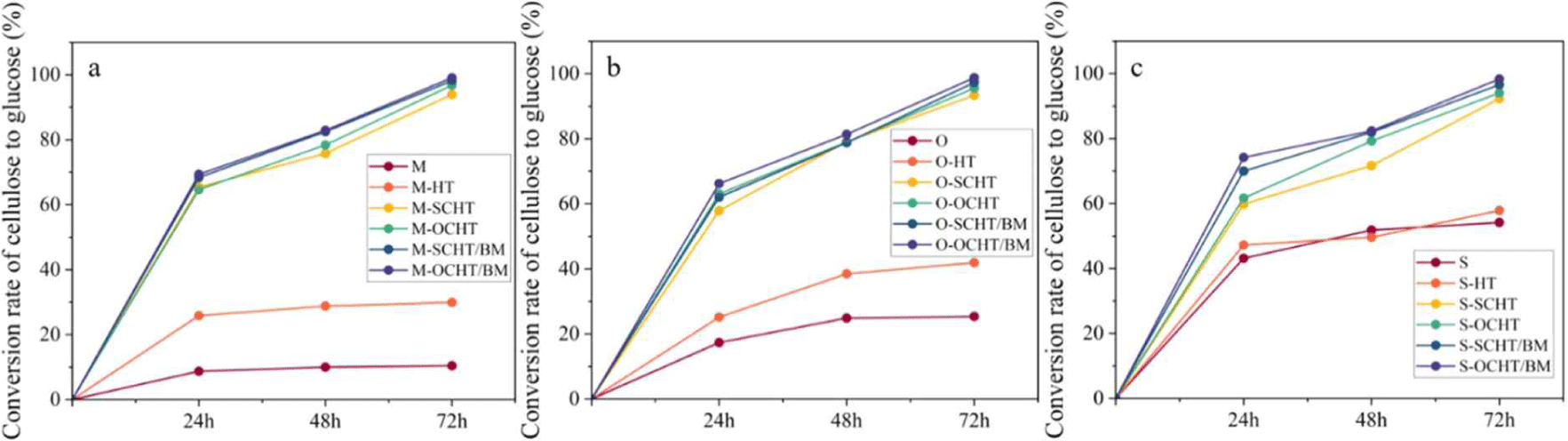
수열처리, 응축수 전처리, 응축수/볼밀 전처리 바이오매스의 효소 흡착 거동은 Langmuir와 Freundlich 등온선 모델로 분석하였으며 결과는 Fig. 4와 Table 2에 나타냈다. Freundlich 등온선 모델의 1/n 값이 0<1/n<1이면 흡착이 유리함을 의미한다. 모든 조건에서 수열처리, 응축수 전처리, 응축수/볼밀 전처리 바이오매스의 1/n 값이 1.85–2.46을 나타내고 있어 효소 흡착 모델을 설명하기에는 적합하지 않다고 판단할 수 있다[21]. Langmuir 등온선을 통해 얻은 분리계수(RL) 값이 0<RL<1이면 흡착 공정이 적합함을 나타내며, 수열처리, 응축수 전처리, 응축수/볼밀 전처리 바이오매스의 분리계수(RL) 값은 0<RL<1을 나타내고 있어 흡착 공정이 적합하다고 판단할 수 있다[22]. 따라서 수열처리, 응축수 전처리, 응축수/볼밀 전처리 바이오매스의 효소 흡착 거동을 설명하기에는 Langmuir model이 적합하며 이는 효소가 바이오매스 표면에 단층 흡착됨을 의미한다. 최대 효소 흡착 용량은 수열처리 대비 응축수 전처리 바이오매스에서 높게 나타났다. 바이오매스 구성성분 분석결과(Fig. 1)를 통해 확인할 수 있듯이 응축수 전처리에 의해 헤미셀룰로오스 분해가 촉진되어 효소의 접근성이 향상된 것이다[1]. 최대 효소 흡착 용량은 M-OCHT/BM에서 67.57 mg/g, O-OCHT/BM에서 66.67%, S-OCHT/BM에서 62.50 mg/g으로 응축수/볼밀 전처리에서 가장 높게 나타났다. 바이오매스 종류에 따른 효소 흡착 용량의 차이는 결정화도, 셀룰로오스의 함량 등 바이오매스 구조와 성분 함량의 차이 때문이다.
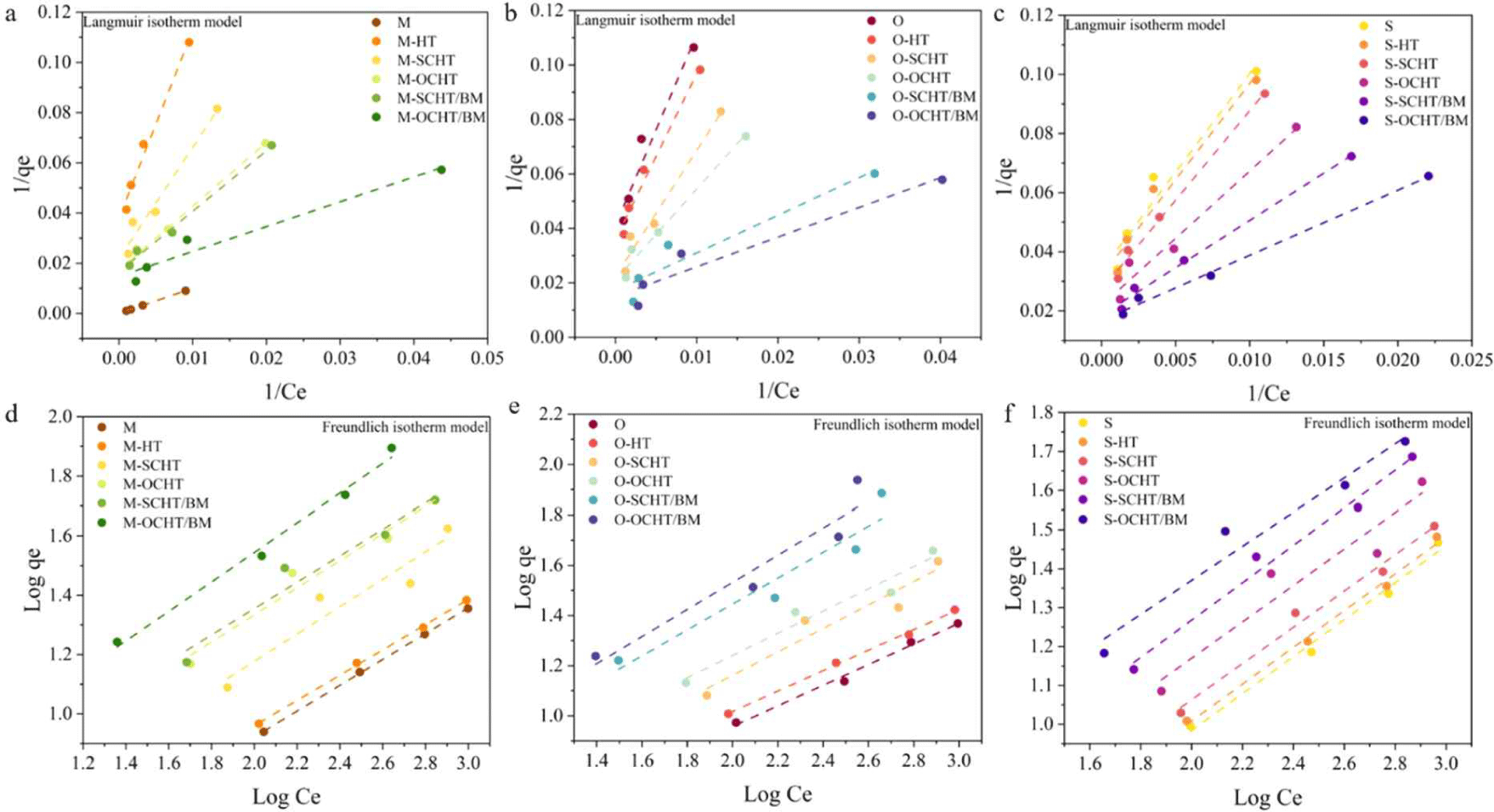
결 론
본 연구에서는 참나무, 거대억새, 단수수 바가스에 대해 수열 처리, 응축수 전처리, 응축수/볼밀 전처리를 수행하였으며, 응축수가 전처리 및 효소가수분해 효율에 미치는 영향을 분석하였다. 수열처리에서 사용된 H2O 대신 응축수로 전처리했을 때 전처리 효과가 향상되었다. 수열처리와 비교하여 헤미셀룰로오스 함량이 감소하였으며, 최종적으로 효소가수분해 효율을 향상시켰다. 또한, 응축수 전처리 후 볼밀을 수행했을 때 바이오매스 결정화도와 입자크기가 감소하여 응축수 단독 전처리에서 보다 높은 효소가수분해 효율을 나타냈다. 이것은 바이오매스의 헤미셀룰로오스 함량 및 셀룰로오스의 결정화도가 효소가수분해 효율에 영향을 미치는 중요한 인자라는 것을 의미한다. 효소의 흡착거동은 Langmuir model로 설명할 수 있으며, 이것은 바이오매스에 효소가 단층 흡착을 의미한다. 따라서 응축수 전처리는 기존의 수열처리를 대신할 수 있으며, 연속적으로 볼밀 전처리를 수행할 경우 바이오매스의 효소가수분해 효율을 향상시킬 수 있다.